CLAIRO Zoom-in 3: Modelling air pollution removal by urban greenery in Ostrava
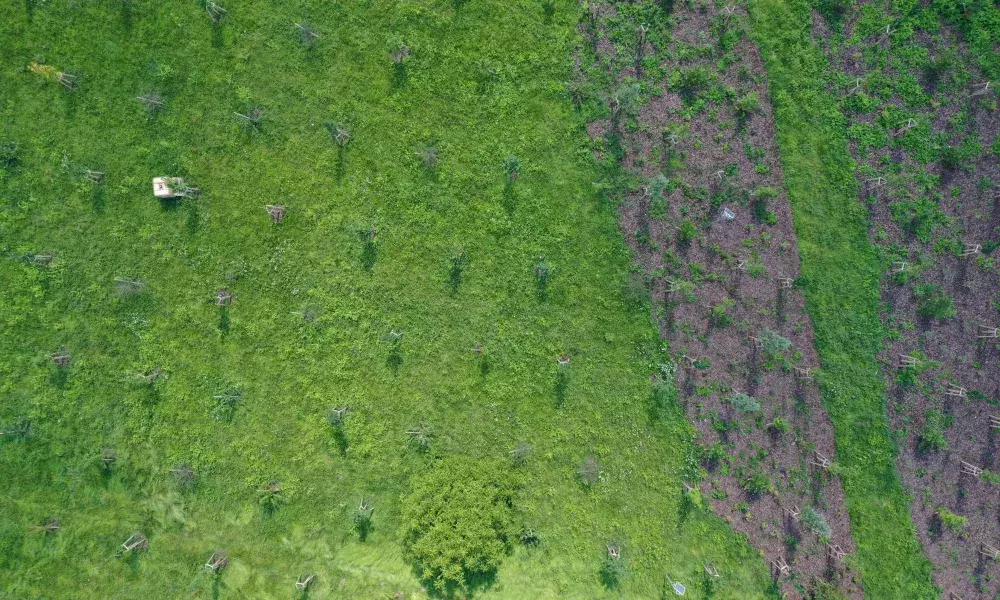
Air pollution, which is the leading environmental cause of mortality globally, kills annually according to the estimations of WHO approximately 7 million people worldwide. The estimates of the researchers of the University of Birmingham and the Harvard University are even darker. In line with their study published in April 2021 over 10 million premature deaths are attributable to fossil fuel combustion alone per year (Vohra et al.,2021), far more than the estimated 1.3 million people who die each year as a result of road traffic accidents. This mortality is mostly due to exposure to fine particulates, but exposure to ozone, nitrogen dioxide and sulphur dioxide is also linked to health risks.
In Europe, a significant proportion of the population lives in areas where pollution poses serious health risks. Around 90% of city dwellers in Europe are exposed to pollutant concentrations that are considered harmful to health.
The City of Ostrava remains one of the air pollution hotspots of Europe despite a major restructuring of the industry and various effective emission control measures, where key pollution sources are industrial plants, traffic, and domestic heating. Two-third of the population of the Ostrava agglomeration feel bothered by poor air quality, and every fourth residents consider relocation due to air pollution. See details here.
Air pollution is a major contributor to chronic diseases, including cardiovascular diseases, chronic obstructive pulmonary disease, asthma and lung disease (Howse et al., 2021). In 2016, according to WHO estimates, globally almost 60% of outdoor air pollution-related premature deaths were due to cardio-vascular diseases, including heart disease and stroke. A much lower proportion, 18% of deaths were due to various respiratory diseases and 6% were attributable to lung cancer.
Fine particulate matter can stay in the bloodstream for several months, damaging the blood vessels and increasing the risk of blood clots, leading to stroke or heart attacks. Even coarse particulates (PM10) can cause various health problems, including high blood pressure, and asthma (Chen et al., 2019).
Ozone pollution can cause coughing and sore throat, can damage the airways, make breathing difficult and aggravate respiratory diseases. Nitrogen dioxide exposure has similar health effects, as it can irritate the airways and can exacerbate respiratory diseases, and particularly asthma.
Moreover, exposure to air pollution was found to be linked to the rise of depression, and to increased risk of dementia (Peters et al., 2019). In addition to adverse health effects, air pollution was found to be associated with higher crime rates. Even moderate pollution was found to increase crime (BBC, 2020).
.
Urban green infrastructure can bring about positive health outcomes through reducing the public’s exposure to air pollution. The use of greenery can offer options for mitigating air pollution that can effectively complement conventional emission control strategies. Vegetation plays an important part in controlling the flow and distribution of pollutants.
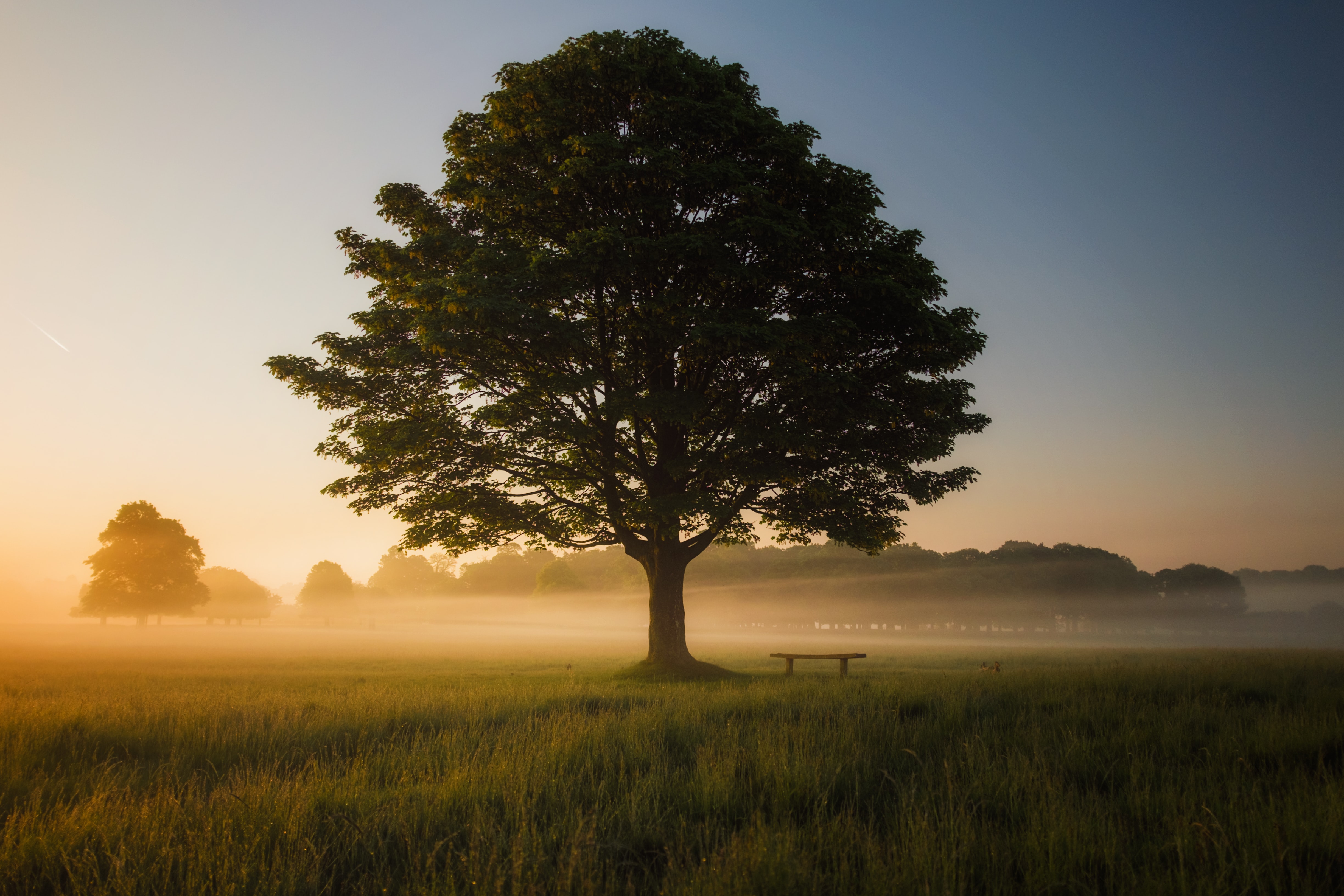
A vegetation barrier, such as a hedge can halve the concentrations of pollutants in its immediate vicinity. The introduction of hedges between road and pedestrians are particularly effective in reducing pollutant concentrations through dispersive effect. Hedges along roadsides can cut back the concentration of black carbon, a key component of fine particulate matter, by up to 63%.
Green infrastructure can reduce the concentration of air pollutants locally by enhancing their deposition rates, since pollutants deposit more efficiently on vegetation than on smoother artificial surfaces (Neft et al., 2016). Trees and shrubs have a relatively high surface area that can function as natural sink for pollutants. The rate of deposition is subject to the available surface area as well as the roughness of the surface (Hewitt et al., 2020).
Urban green spaces can even help in reducing emissions indirectly, through taking the place of emission sources, or through encouraging less polluting active transport.
The extent to which various plant species perform pollutant removal depends on a number of characteristics, such as the surface and shape of the canopy, vegetation density, the longevity of plant leaves, the size of the leaves, or the characteristics of the leaf surface. Details are provided in an article on ‘Designing greenery for pollutant capture under CLAIRO’.
Capture efficiency is not the only criteria that needs to be born in mind during the selection of plant species for supporting pollutant removal. Greenery can also be a source of pollution. Trees and other plants produce volatile organic compounds, which when exposed to sunlight react with nitrogen oxides emitted mainly from vehicles and industrial sources to form ozone, which can lead to the formation of ground-level smog. In addition, many tree species produce pollen, which can cause allergic reactions. (Barwise et al., 2020) When planning urban greenery in the vicinity of residential areas, it is wise to avoid planting tree species producing highly allergenic pollen, such as birch, ash, oak, and elm, or those ones that emit significant amount of volatile organic compounds, such as black locust, poplar or plane tree (Gallis, 2020).
Plants will filter effectively air pollutants only as long as they can grow properly and stay healthy. In urban environments vegetation has to cope with multiple stressors, such as lack of root space, soil compaction, lack of oxygen, nutrients and water, poor air quality or salt pollution. Considering these extreme conditions in urban systems, when assessing the suitability of various species, it is plants’ stress tolerance that should be given priority over all other functionalities (Tiwary et al., 2015). In addition to stress tolerance, adaptability to local topographical, soil and climatic conditions should be taken into account. The ecological relationships of individual species should also be considered. Milos Zapletal, the leader of the research team responsible for greenery design under CLAIRO at the Silesian University in Opava highlighted that
Plant diversity is also a decisive factor. Greater species diversity leads to greater ecosystem health and resilience to environmental stresses.
The detailed design of the greenery structure and composition for the target sites of CLAIRO undertaken by Milos Zapletal, Pavel Samec and Vit Kaspar from the Silesian University reflected all these factors. The greenery design in the City of Ostrava was largely based on the methodology for plant species selection process for improved air quality developed by Barwise and Kumar in the study titled, ‘Designing vegetation barriers for urban air pollution abatement: a practical review for appropriate plant species selection’. The main steps of the selection process are summarized in Figure 1.
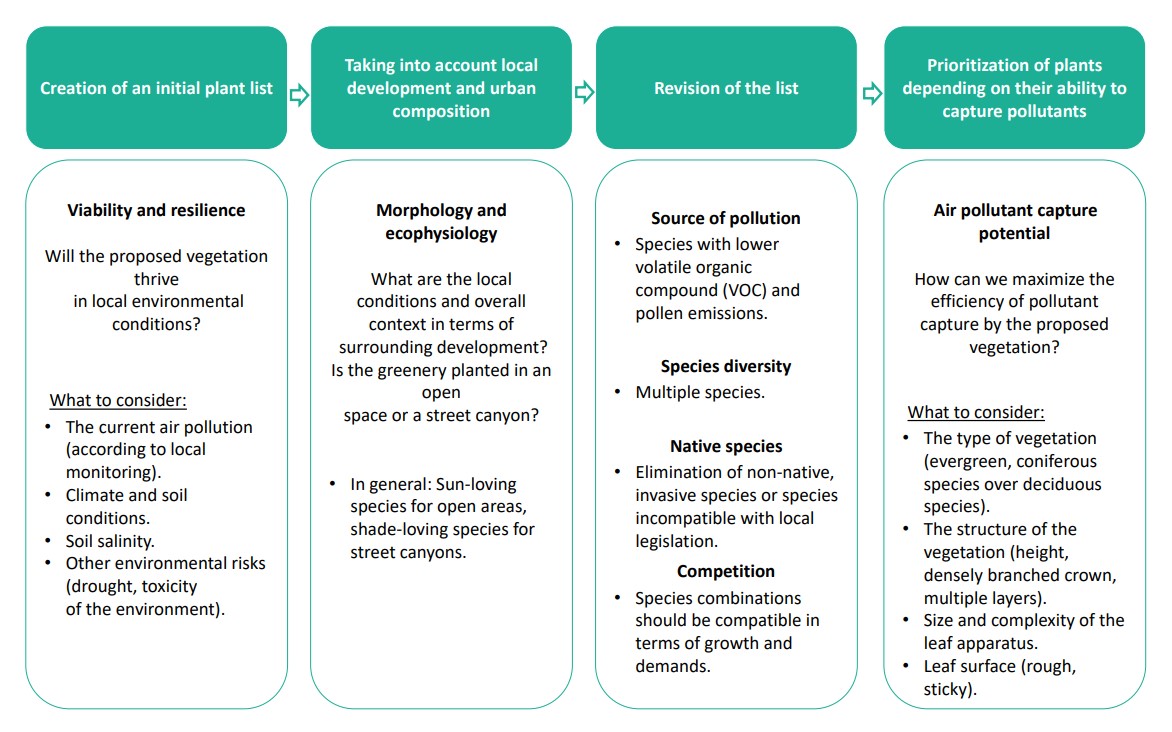
In line with the method at first a longer initial plant list was created with suitable candidate species, then during the assessment of main aspects less appropriate species were removed from the base list. First it was evaluated whether the species could grow well in local environmental conditions, such as microclimate, soil conditions, the quality of the air, or soil salinity, and subsequently the local urban settings (i.e., available space, local light conditions), and then factors influencing species diversity and ecological links were studied.
As a result of the targeted design, multi-layer tree and shrub communities were planned in Ostrava for the two target sites of CLAIRO to maximize capture efficiency. Exclusively native tree species were selected for planting. During the selection process, native and uncultivated plant forms were preferred.
As a final step, from the resulting streamlined list, species with the greatest air pollutant capture potential were selected.
To support cities in cutting back pollution exposure across Czech Republic and beyond an online database of plants was created by the Silesian University in Opava under CLAIRO. The database is containing over 150 tree species presenting higher resistance to air pollution and higher pollutant capture efficiency. The listed trees are classified according to the type of leaf apparatus, climatic requirements, preferred soil type, soil moisture needs, and sensitivity to acid deposition, tropospheric ozone and particulate matter.
As part of the CLAIRO project, a model of capture of air pollutants was developed by Milos Zapletal and Vit Kaspar from the Silesian University in Opava on the basis of a couple of standard models for the deposition of pollutants. Initially the focus was on simple deposition models, such as UFORE, also the model created by Fang and Wu, as well as the one by Wesely. Later on, during the project’s implementation period, the team of the Silesian University gradually started to use more sophisticated models, such as the one developed by Zhang and the one created by Emberson.
The Urban Forest Effects (UFORE) model developed by David J. Nowak and Daniel E. Crane for the Forest Service of the United States Department of Agriculture aims to quantify urban forest structure and functions, using standard field data, air pollution and meteorological data. UFORE has five model components linked among others to the anatomy of urban forest, emissions of volatile organic compounds by vegetation, and carbon storage and sequestration. One of the five model components, UFORE-D quantifies the amount of pollution removed by the urban forest and evaluates the improvement in air quality throughout a year. Pollution removal is calculated for O3, SO2, NO2, CO, and PM10. In the model the concentration of compounds and their deposition rate are used to estimate the deposition of various air pollutants. For the quantification of capture by greenery the amount of leaf material area in a canopy is also necessary that is described by the leaf area index (LAI).
In Taiwan Guor-Cheng Fang and Yuh-Shen Wu developed a deposition model for particulates that take into account particle settling. In the settling of particulate matter atmospheric turbulence plays a major role that is influenced by surface roughness and wind speed. (Noll and Fang, 1989; Fang et al, 1999).
For gaseous deposition Marvin L. Wesely developed a model in the United States in the late eighties. Plant response in this method is determined through a ‘meteorological approach’ rather than the direct simulation of the physiology of plants offering a significantly reduced computational time. One of the drawbacks of this model is that variations of characteristics by individual plant species are not considered explicitly (Wesely, 1989).
As the above models were not sufficiently differentiated for variations in vegetation types leading to inaccuracy in the long-term predictions of pollutant capture, they were replaced over time by more complex models under CLAIRO.
Among the more complex models used, the one developed by a Canadian research team led by Leiming Zhang seeks to evaluate particle dry deposition. It addresses the various stages of the deposition processes, such as, turbulent transfer, impaction, gravitational settling, and particle rebound. The main improvement offered by this model compared to earlier ones is that it produces more accurate estimations for sub-micron particles. (Zhang et al., 2001)
The other more sophisticated model applied by the Silesian University under CLAIRO was developed by an international research team led by Lisa Dianne Emberson for modelling the process of ozone deposition. The use of the model enables the prediction of seasonal and daily ranges in deposition rates. (Emberson et al., 2001) As opposed to previous models, this method is able to simulate the effect of a number of key parameters on the uptake of ozone by stomata, which are small pores on the leaf surfaces controlling gas exchange. These parameters include vegetation properties, solar radiation, temperature, and the amount of moisure in the air and soil. With the use of the model, it is possible to estimate stomatal ozone uptake for a number of plant species. The amount of moisture in the air and soil are also key parameters, as dry conditions can substantially limit stomatal ozone uptake as a result of stomatal closure. (Emberson et al., 2000)
The CLAIRO model is meant to enable the accurate quantification of the positive effects of greenery on air quality. During the quantification of capture, the deposition of the various pollutants is estimated based on the concentration of compounds and their deposition rate. Concentration values were provided by sensors. For the quantification of capture by greenery, apart from the estimation of deposition, the calculation of the leaf area index is also necessary. Below a simplified diagram is presented on the model of pollutant capture by green infrastructure (Figure 2).
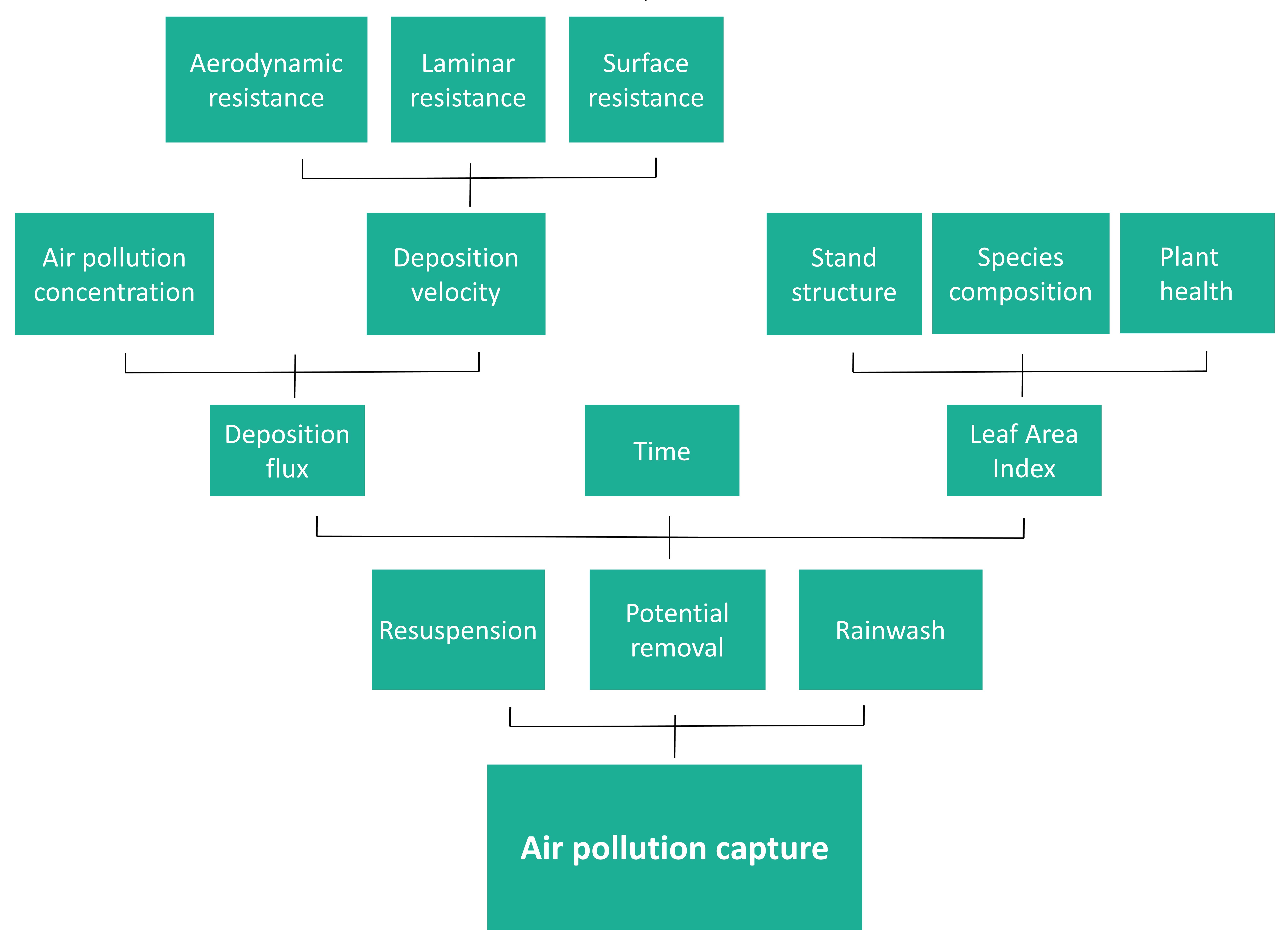
A guide on the CLAIRO Methodology targeting at air quality and urban green infrastructure experts and the research community and its shorter version the CLAIRO Manual were made available providing details about the greenery design and the model of CLAIRO. The English versions of these documents were published in early 2022.
During project implementation the use of different approaches were tested for the calculation of leaf area index (LAI), which is a crucial parameter describing vegetation properties that directly affects deposition rate.
Initially, as part of the assessment of the original vegetation, for the determination of the leaf area index the tree species were inventoried. During field inventory ground-based measurements were undertaken: canopy heights were measured using a digital altimeter, crown diameters of trees were determined, and the health status of the plants was also assessed as it directly influences leaf area.
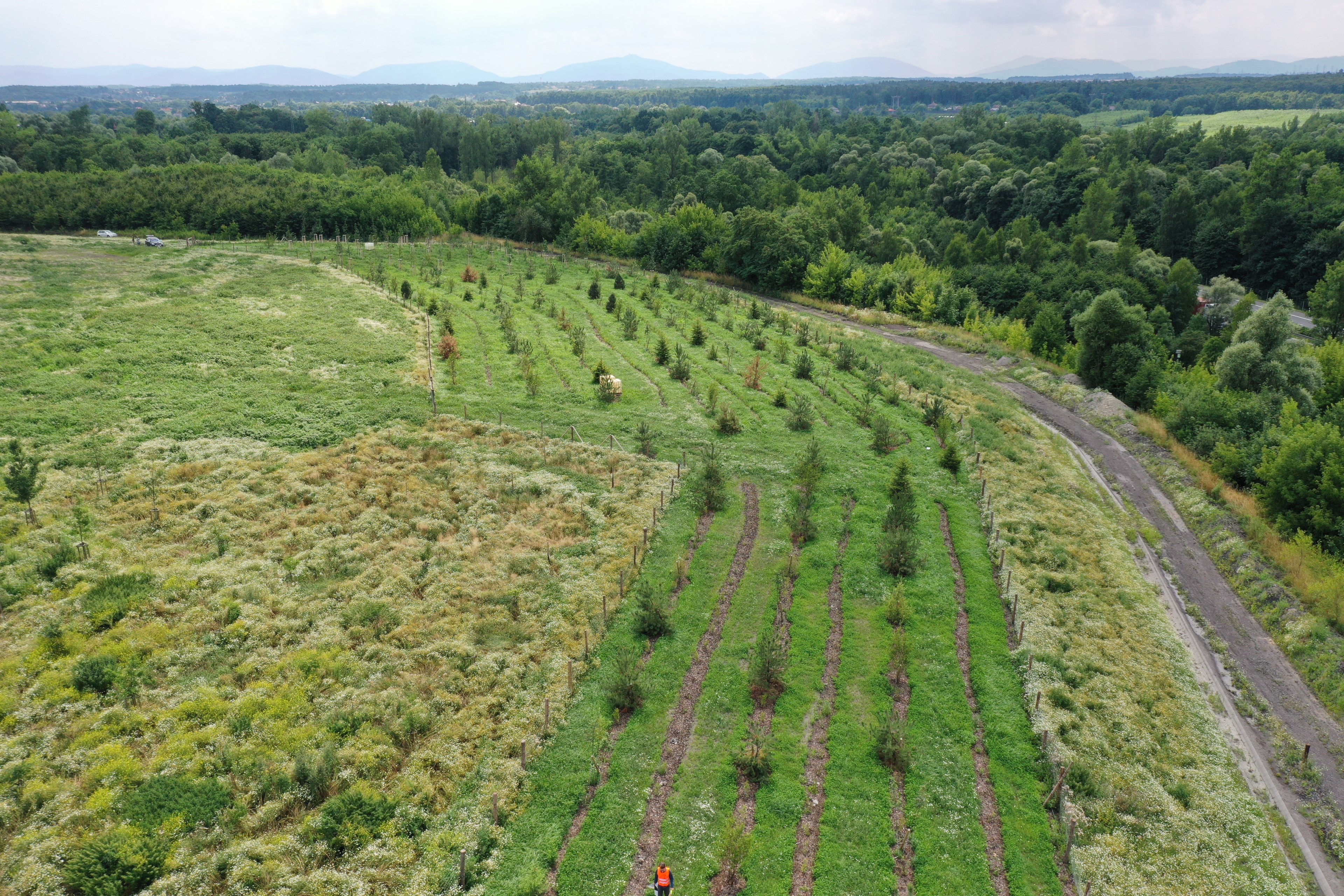
Explaining the deficiencies of standard approaches aiming at the quantification of deposition and pollutant capture, Vit Kaspar from the Silesian University in Opava highlighted that during the development of the model of CLAIRO:
The question was how to distinguish between different vegetation types and different life stages. Because we had trees, we had shrubs, and grass, and we also had deciduous trees, and conifers. And the goal was to get differentiated information on species level. What is actually the deposition on an oak tree?
The problem was that the models available for dry deposition are rather simplified, using land-cover type as input parameter (for instance forest or arable field in general), but no differentiated data is foreseen for coniferous and deciduous trees.
Therefore, following planting, with an aim to test and demonstrate a novel and more advanced approach, drone-based data was used for LAI calculation. Vit Kaspar pointed out that
Drone-based measurement represents a transition between the use of in-situ or ground-based data and satellite data.
The overall goal was to distinguish the effects of various plant species and life stages of vegetation on pollution capture through deposition.
The flight mission of the drone was carried out in July 2021 at a flight altitude of 70-80 m above ground. This period was selected intentionally, as it is the peak of the growing season, when canopies have dense foliage.
Drones were used in combination with hemispherical photography for modelling air pollution removal. Hemispherical upward looking photographs are made by using wide-angle lens that allow a 360° view of the canopy from the earth's surface. The individual pixels are divided into classes, one of which represents the sky, while the other represents physical obstacles, such as leaves, branches, tree trunks, and the surrounding terrain that prevent the penetration of sunlight. The ratio of these two classes determines the total coverage of the tree canopy, from which leaf area index can be calculated. (Fournier and Hall, 2017)
Hemispherical photographs were taken at several points within the study area. Then the data from the drone in combination with the point values sourced from hemispherical photographs were scaled up into a continuous map.
The use of drones for the quantification of vegetation properties offers a range of benefits:
- it provides finer spatial resolution and ensures higher level of accuracy than the application of field inventory,
- this approach is less time-consuming and laborious than the application of field inventory,
- flight missions can be repeated easily,
- it allows weather-based timing.
Most importantly, drone-based measurement allows capturing local-scale in greater detail, as well as seasonal variations of the vegetation.
It was demonstrated by the model applied in Ostrava that per plant cover mature trees had the highest removal rate, due to their higher leaf area. In contrast, the lowest removal rates were calculated for tree saplings, owing to their lower leaf area.
The CLAIRO project provided substantial support to European cities aiming at improving urban air quality with the use of urban green spaces, through making available a package of useful tools: a universal method helping greenery design, an online database of plant species with higher pollutant capture efficiency, and a model of pollutant removal.
The green infrastructure design developed by the Silesian University for the target sites of CLAIRO in Ostrava defined the most appropriate greenery structure and deposition to maximize air pollutant removal resulting in multi-layer tree and shrub communities.
The model that was put in use under CLAIRO has vital role in verifying the effectiveness of the greenery design, as it enables the prediction of the magnitude of pollutant capture for the upcoming years. The estimation of plant efficiency in the filtration of air pollutants is quite complicated because it depends on a high number of factors. A model of pollutant capture that was applied under CLAIRO was meant to simplify the evaluation process and at the same time sought to provide accurate estimations. The opportunity for accurate predictions is crucial, since decades are required for a new plantation to become a mature forest, that can effectively filter air pollutants, while only a couple of years were dedicated to project implementation. Accuracy was ensured by applying model components that allowed species-specific simulation of pollutant removal.
CLAIRO enabled the testing and demonstration of the applicability of an innovative practice based on drone-based measurements of vegetation properties in the Ostrava target sites. The approach based on a combination of drone-based data collection and hemispherical photography enabled the modelling species-specific differences and seasonal variations of the vegetation that influence the rate of deposition. Positive experiences with the relatively low-cost drone-based measurements in Ostrava can open the door for other cities for accurate optimization of urban vegetation for pollutant removal.
Barwise, Y., Kumar, P. (2020) Designing vegetation barriers for urban air pollution abatement: a practical review for appropriate plant species selection. npj Clim Atmos Sci 3, 12. https://doi.org/10.1038/s41612-020-0115-3.
BBC. 'How air pollution is killing us' https://www.bbc.com/future/article/20190415-how-air-pollution-is-doing-more-than-killing-us
BBC. (2020) ‘The best trees to reduce air pollution’. https://www.bbc.com/future/article/20200504-which-trees-reduce-air-pollution-best
British Heart Foundation. ‘Heart attack and stroke deaths related to air pollution could exceed 160,000 by 2030’ https://www.bhf.org.uk/what-we-do/news-from-the-bhf/news-archive/2020/january/heart-and-circulatory-deaths-related-to-air-pollution-could-exceed-160000-over-next-decade
Chen, CH. et al. (2019) The effects of fine and coarse particulate matter on lung function among the elderly. Sci Rep 9, 14790. https://doi.org/10.1038/s41598-019-51307-5
EEA. ‘Air pollution’. https://www.eea.europa.eu/themes/air/intro
EEA. ‘Premature deaths attributable to air pollution’ https://www.eea.europa.eu/media/newsreleases/many-europeans-still-exposed-to-air-pollution-2015/premature-deaths-attributable-to-air-pollution
Emberson L.D., Ashmore M.R., Cambridge H.M., Simpson D, Tuovinen J.P. (2000) Modelling stomatal ozone flux across Europe. Environ Pollut. 2000 Sep;109(3):403-13. doi: 10.1016/s0269-7491(00)00043-9. PMID: 15092873.
Emberson, L.D., Ashmore, M., Simpson, D. et al. (2001) Modelling and Mapping Ozone Deposition in Europe. Water, Air, & Soil Pollution 130, 577–582. https://doi.org/10.1023/A:1013851116524.
EPA. ‘Basic Information about NO2’ https://www.epa.gov/no2-pollution/basic-information-about-no2
EPA. ‘Health Effects of Ozone Pollution’ https://www.epa.gov/ground-level-ozone-pollution/health-effects-ozone-pollution#:~:text=Ozone%20can%20cause%20the%20muscles,and%20sore%20or%20scratchy%20throat.
Fang GC, Wu YS, Chang CN, Chang KF, Yang DG (1999) Modeling dry deposition of total particle mass in trafficked and rural sites of Central Taiwan. Environ Int 25(5–25):625–633. doi:10.1016/ S0160-4120(99)00021-5
Fournier, R., Hall, R. (2017) Hemispherical Photography in Forest Science: Theory, Methods, Applications. Managing Forest Ecosystem. Springer: 313.
Gallis, C., Shin, WS. (2020) Forests for public health. Cambridge Scholars Publishing, ISBN (10) 1-5275-5029-X.
Healthline. ‘Pollen Library: Plants That Cause Allergies’ https://www.healthline.com/health/allergies/pollen-library#Grass-Pollen-Allergies
Hewitt, C.N. et al. (2020) Using green infrastructure to improve urban air quality (GI4AQ). Ambio 49, 62–73. https://doi.org/10.1007/s13280-019-01164-3.
Howse, H., et al. (2021) Air pollution and the noncommunicable disease prevention agenda: opportunities for public health and environmental science. Environmental Research Letters, Vol. 16, 065002.
IES. ‘Role of trees & other green infrastructure in urban air quality’ https://www.the-ies.org/analysis/role-trees-and-other-green
i-Tree. https://www.itreetools.org/documents/53/UFORE%20Methods.pdf
Janhäll, S. (2015) Review on urban vegetation and particle air pollution - deposition and dispersion. Atmos. Environ. 105, 130-137, ISSN 1352-2310, https://doi.org/10.1016/j.atmosenv.2015.01.052.
Neft, I. et al. (2016) Simulations of aerosol filtration by vegetation: Validation of existing models with available lab data and application to near-roadway scenario. Aerosol Science and Technology, 50: 937–946. https://doi.org/10.1080/02786826.2016.1206653.
Noll KE, Fang KYP (1989) Development of a dry deposition model for atmospheric coarse particles. Atmos Environ 23(3):585–594
Nowak, D. J. (1994). “Atmospheric carbon dioxide reduction by Chicago's urban forest,” in Chicago's Urban Forest Ecosystem: Results of the Chicago Urban Forest Climate Project. General Technical Report NE-186, eds McPherson, E. G., Nowak, D. J., Rowntree R. A. (Chicago, IL: US Department of Agriculture, Forest Service), 83–94.
Nowak, D. J. (2000). The Effects of Urban Trees on Air Quality. USDA Forest Service, Syracuse, NY, 1–4.
Peters, R. et al. (2019) Air Pollution and Dementia: A Systematic Review. J Alzheimers Dis. 2019;70(s1):S145-S163. doi: 10.3233/JAD-180631. PMID: 30775976; PMCID: PMC6700631.
Silva, S.J., Heald, C.L. (2017) Investigating Dry Deposition of Ozone to Vegetation. https://doi.org/10.1002/2017JD027278
The Guardian. ‘Small increases in air pollution linked to rise in depression, finds study’ https://www.theguardian.com/environment/2020/oct/24/small-increases-in-air-pollution-linked-to-rise-in-depression-finds-study
Tiwary, A., Williams I.D., et al. (2015) Development of multi-functional streetscape green infrastructure using a performance index approach. Environmental Pollution, 208(Pt A):209-220, doi: 10.1016/j.envpol.2015.09.003.
University of Surrey. ‘Plant hedges to combat near-road pollution exposure’ https://www.surrey.ac.uk/news/plant-hedges-combat-near-road-pollution-exposure
Vohra, K. et al. (2021) Global mortality from outdoor fine particle pollution generated by fossil fuel combustion: Results from GEOS-Chem. Environmental Research, Vol. 195, 110754, ISSN 0013-9351, https://doi.org/10.1016/j.envres.2021.110754.
Wesely, M.L. (1967) Parameterization of surface resistances to gaseous dry deposition in regional-scale numerical models. Atmospheric Environment, Vol. 23, Issue 6, 1989, pp 1293-1304, ISSN 0004-6981.
WHO. ‘Air pollution’. https://www.who.int/health-topics/air-pollution#tab=tab_1
WHO. ’Ambient (outdoor) air pollution’. https://www.who.int/news-room/fact-sheets/detail/ambient-(outdoor)-air-quality-and-health
WHO. ‘Road traffic injuries’. https://www.who.int/news-room/fact-sheets/detail/road-traffic-injuries
Zapletal, M., Cudlín, et al. (2011) Ozone flux over a Norway spruce forest and correlation with Net Ecosystem Production. Environmental Pollution, 159, 1024-1034.
Zhang, L., Gong, S. et al. (2001) A size-segregated particle dry deposition scheme for an atmospheric aerosol module. Atmospheric Environment, Vol. 35, Issue 3, 2001, pp. 549-560, ISSN 1352-2310, https://doi.org/10.1016/S1352-2310(00)00326-5.
About this resource
The Urban Innovative Actions (UIA) is a European Union initiative that provided funding to urban areas across Europe to test new and unproven solutions to urban challenges. The initiative had a total ERDF budget of €372 million for 2014-2020.
Similar content
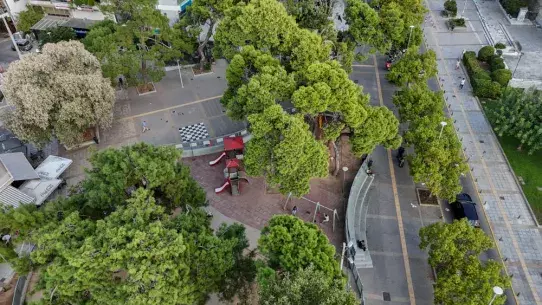
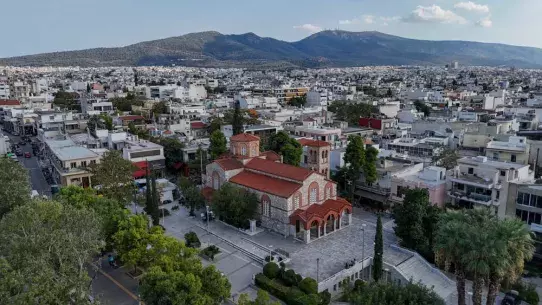